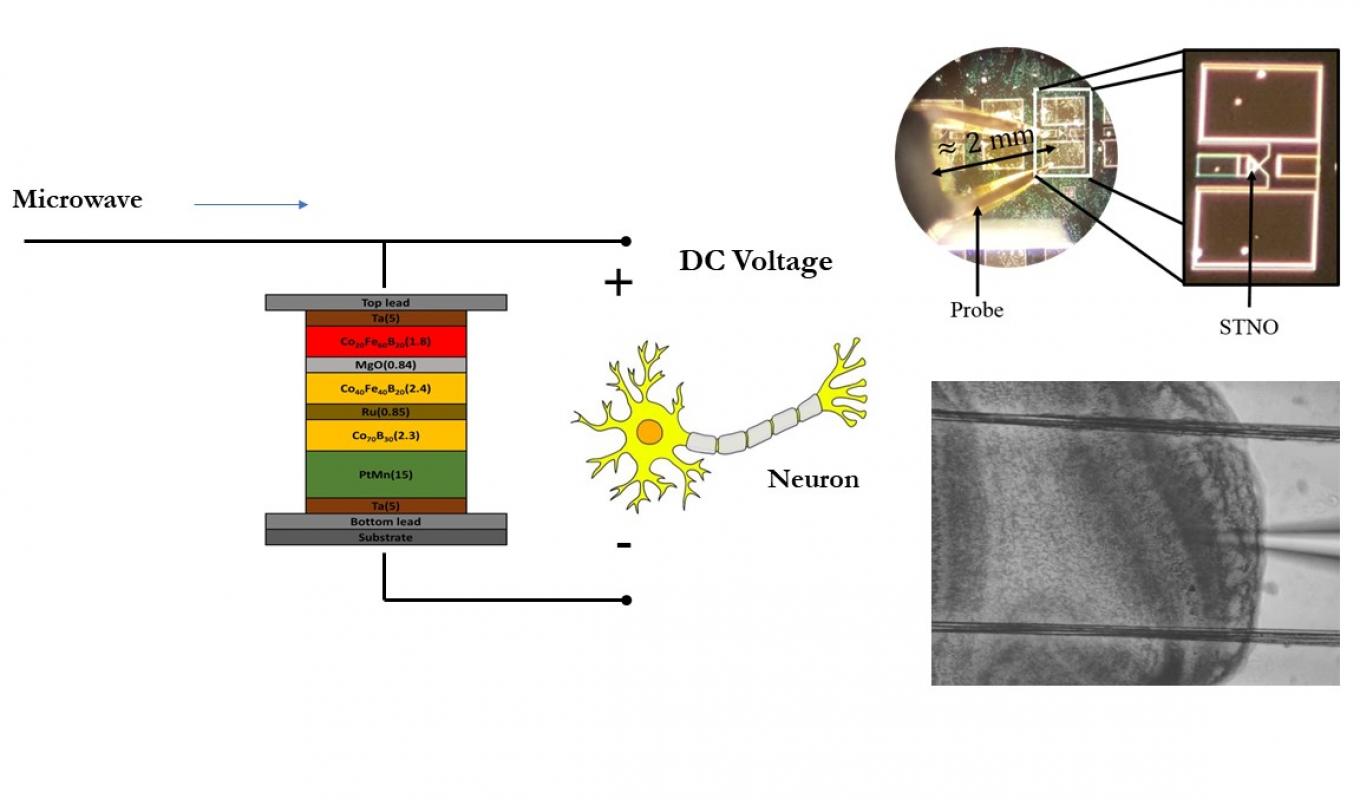
The brain is a complex network of interconnected circuits that exchange signals in the form of action potentials. These action potentials hold the key to understanding how the brain processes information and generates complex thought. Currently, the most advanced method for performing highly localized measurements of neuronal action potentials in humans and other primates requires implanting electrodes to target areas of the brain. In humans, this method can only be used in conjunction with other clinically-indicated procedures, (e.g., surgical tumor removal), and is therefore not possible in normal subjects. Optical methods based on voltage sensitive contrast agents (dyes, quantum dots) have also been demonstrated. However, focused optical beams cannot penetrate the skull or deep tissue, and thus cannot access deep-brain regions. In contrast to light, microwave frequency signals can safely and efficiently penetrate through deep tissue and the skull, and are thus ideal for studying the inner workings of the brain. An important example of microwave-enabled study is functional magnetic resonance imaging (fMRI), which has shed tremendous insight on human cognition. However, fMRI cannot directly measure electrical action potentials. Rather, it detects blood oxygenation status which is an indirect and slow measure of brain activity. Furthermore, fMRI cannot temporally resolve individual neuronal activations or spatially resolve small groups of neurons, and thus can only study collective effects in large areas of the brain. The ability to non-invasively detect localizes currents from individual neurons or small clusters remains one of the grand challenges of neuroscience, and is essential to understand the inner workings of the brain. We are developing a novel approach for non-invasive measurements to read out action potentials across the whole brain from single neurons. We take advantage of recent advances in nanoscale spintronic devices to create injectable nano-reporters that measure and control weak electrical signals in the brain wirelessly. This approach would address one of the biggest challenges facing neuroscience today: the ability to measure and control the activity of single neurons non-invasively deep in the brain.