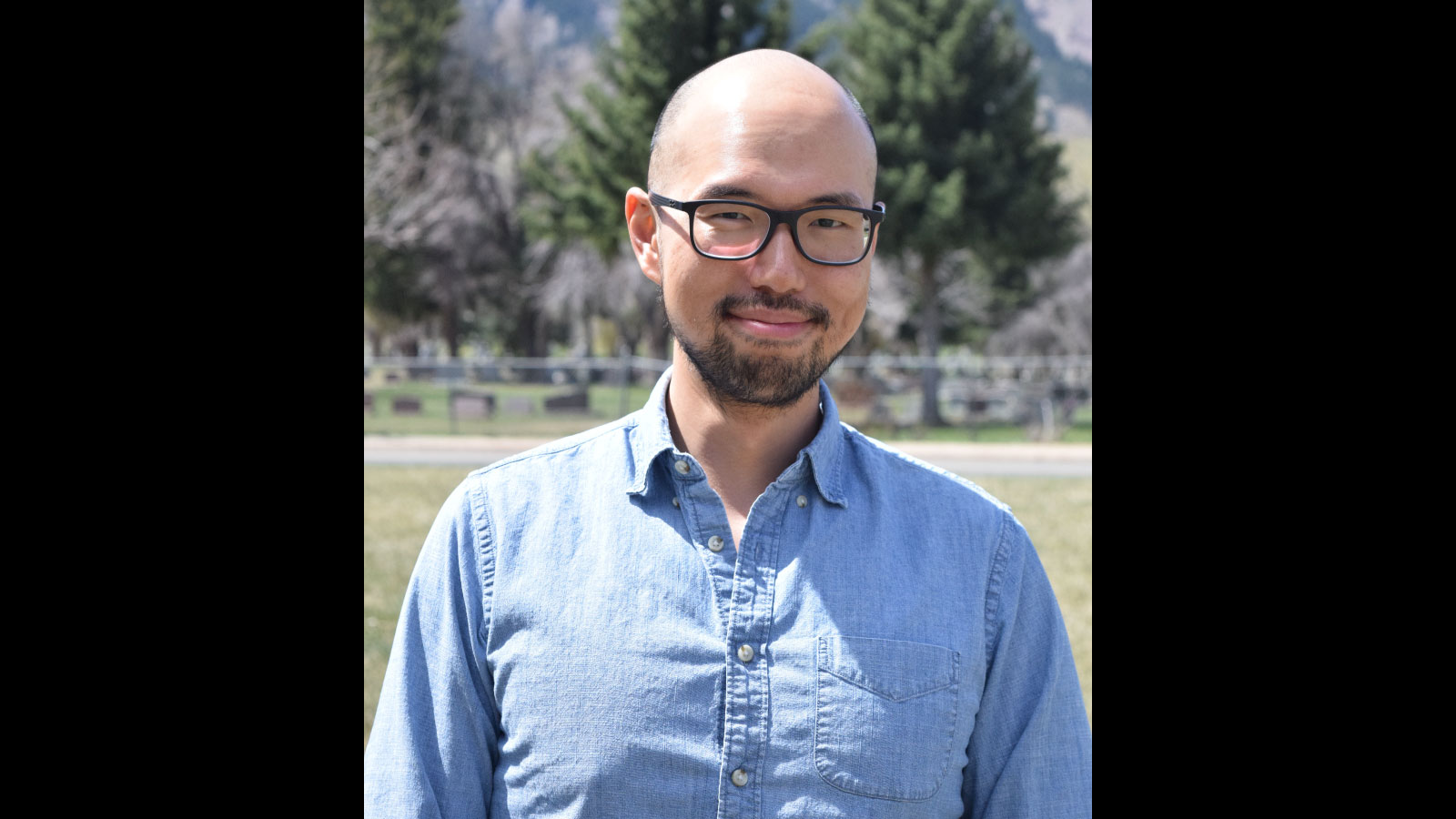
Yu Liu. (Credit: Margie Bruff)
Lasers are often misunderstood, according to chemical physicist Yu Liu, one of JQI’s newest Fellows.
“People usually think of lasers as heating things up, but if you use the right frequency of lasers and target the right type of atoms, you can actually take energy away,” Liu says.
This technique, known as laser cooling, is Liu’s specialty. He built his career on cooling molecules to “ultracold” temperatures—a mere thousandth or millionth of a degree above absolute zero—to observe their reactions in quantum chemistry’s version of slow motion. By slowing down the action and studying a chemical reaction as it plays out, his research and techniques provide insights into the quantum underpinnings of chemistry.
In January 2024, Liu brought his quantum approach to chemistry research to the University of Maryland (UMD) as an assistant professor in the Department of Chemistry and Biochemistry. He set up his lab below ground in the new Chemistry Building—a desirable location since it is more isolated from vibrations and electromagnetic and radio frequency interference than the labs with better views. In the fall, he became a JQI Fellow.
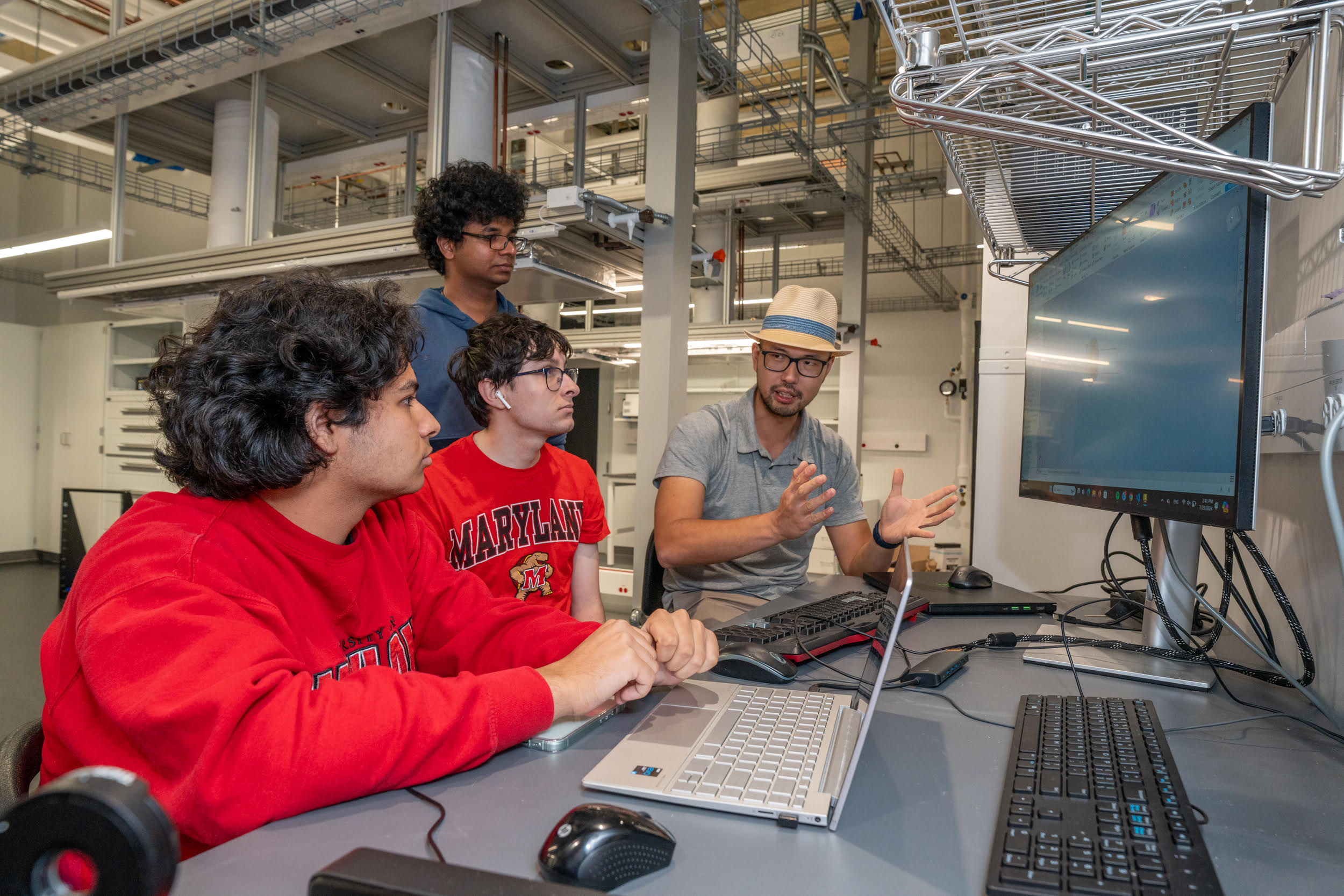
"We at JQI are very excited to include a faculty member from chemistry into our ranks,” says JQI Co-Director Jay Sau. “We hope to see the breadth of membership of JQI continue to increase as a testament to the broader utility of coherent complex quantum science, which is at the intellectual foundation of JQI."
Liu is excited to continue his work as part of UMD’s interdisciplinary community of quantum scientists. UMD has an abundance of quantum centers and institutes whose researchers have created a community that fosters collaborations on diverse quantum research projects and has made the area one of the world’s top quantum research locations.
“The central theme of my research is to apply tools from quantum science to the studies of molecular structures and chemical reactions,” says Liu. “To this end, becoming a member of JQI, one of the world's leading quantum centers, is highly valuable for my research program. Conversely, I hope to leverage my interdisciplinary background in both AMO physics and physical chemistry to help foster synergy between quantum and chemical sciences at UMD.”
Liu’s research has used his training in physics and interest in how chemistry produces the materials that make up our world to make advancements in both fields. Before coming to UMD, he spent three years working on controlling the quantum states of single molecular ions as a postdoctoral researcher at the National Institute of Standards and Technology (NIST) in Boulder, Colorado.
“His research has a depth and fearlessness that reflects his multidisciplinary training in physics and chemistry,” says Janice Reutt-Robey, chair of the Department of Chemistry and Biochemistry.
Watching Molecules Dance
Liu has loved chemistry ever since he was a kid, but he decided to pursue physics in college to gain a deeper understanding of the rules that shape the universe. He earned a bachelor’s degree in physics from the University of Nevada, Las Vegas in 2014 and a Ph.D. in physics from Harvard University in 2020.
While pursuing his Ph.D., Liu studied under Kang-Kuen Ni, who Liu describes as an ultracold molecule “pioneer” and the first person to show him the vast potential of applying physics techniques to chemistry problems. Liu enjoyed the work so much that he focused his dissertation on the reaction dynamics of ultracold molecules—and he’s been pursuing the topic ever since.
“It combined several of my interests and seemed like the perfect project for me at the time,” Liu says. “Having done it for six years now, I’ve really developed a passion for it.”
Liu has already made significant contributions to his field of research. At Harvard, he co-led a 2020 study in the journal Nature Physics on the ability of laser cooling to extend the life of molecules and ions that exist in the middle of a chemical reaction, before the reactants settle into their final products. Cooling everything down enabled researchers to observe the behavior of the molecules over the span of a microsecond—much longer than is possible at room temperature.
“When you have two molecules colliding in a typical chemical reaction, they're going to stick together for about one vibrational rotational period and then they separate,” Liu says. “But in the reaction I was looking at, they come together, vibrate millions of times and keep dancing around each other before they separate.”
However, the research team discovered a drawback: When two ultracold molecules collide and interact, they can be “excited” by the laser that is used to confine them, causing them to heat up and possibly escape the experiment. These findings helped crack the mystery of why ultracold molecules are sometimes lost during experiments. Previously, the loss of molecules plagued researchers, and identifying the laser as the culprit points to ways to mitigate this loss and improve the applicability of ultracold molecules in quantum science and technology.
Liu was also instrumental in developing a technique to observe the quantum states of chemical reaction products. In a paper published in the journal Nature in 2021, he used the technique to study chemical reactions between molecules trapped by lasers at temperatures close to absolute zero.
“By cooling things down, we’re controlling the number of states that these chemical reactions can occupy, and that makes it possible to look at them in extreme detail,” Liu says. “We can tell you how likely it is that a reaction will go to any of these product quantum states—for example, a 10% probability of two products coming out of the reaction rotating in a particular way."
During Liu’s postdoctoral work at NIST, he focused on observing and manipulating just a single molecular ion instead of studying interacting molecules. In a paper he and his colleagues published in Science, they describe an experiment where they fought the natural tendency of a molecule—like all other cold objects—to warm up to the temperature of its surroundings.
In the experiment, the team used lasers to contain a molecule and isolate it in an ultrahigh vacuum setup. Even in the ultrahigh vacuum, the molecule still tended to warm up over time, primarily due to the light that could reach it from the room temperature surroundings.
To keep the molecule cold, Liu and his colleagues didn’t take the most direct cooling approach of constantly pumping energy away like a kitchen freezer. Instead, they demonstrated more finesse and worked to keep it in a particular quantum state. They monitored the molecule for any changes and then hit it with microwaves to reverse any changes they observed. To monitor the molecule, they used an atomic ion that was held in the same ion trap. The electric charge of the atom and molecule make them tug on each other and allowed the researchers to detect the quantum state of the molecule while only needing to interact with the simpler atom that is more convenient for them to manipulate. Using the technique increased the amount of time the molecule spent in the desired state by about a factor of 20.
Liu Lab’s Projects
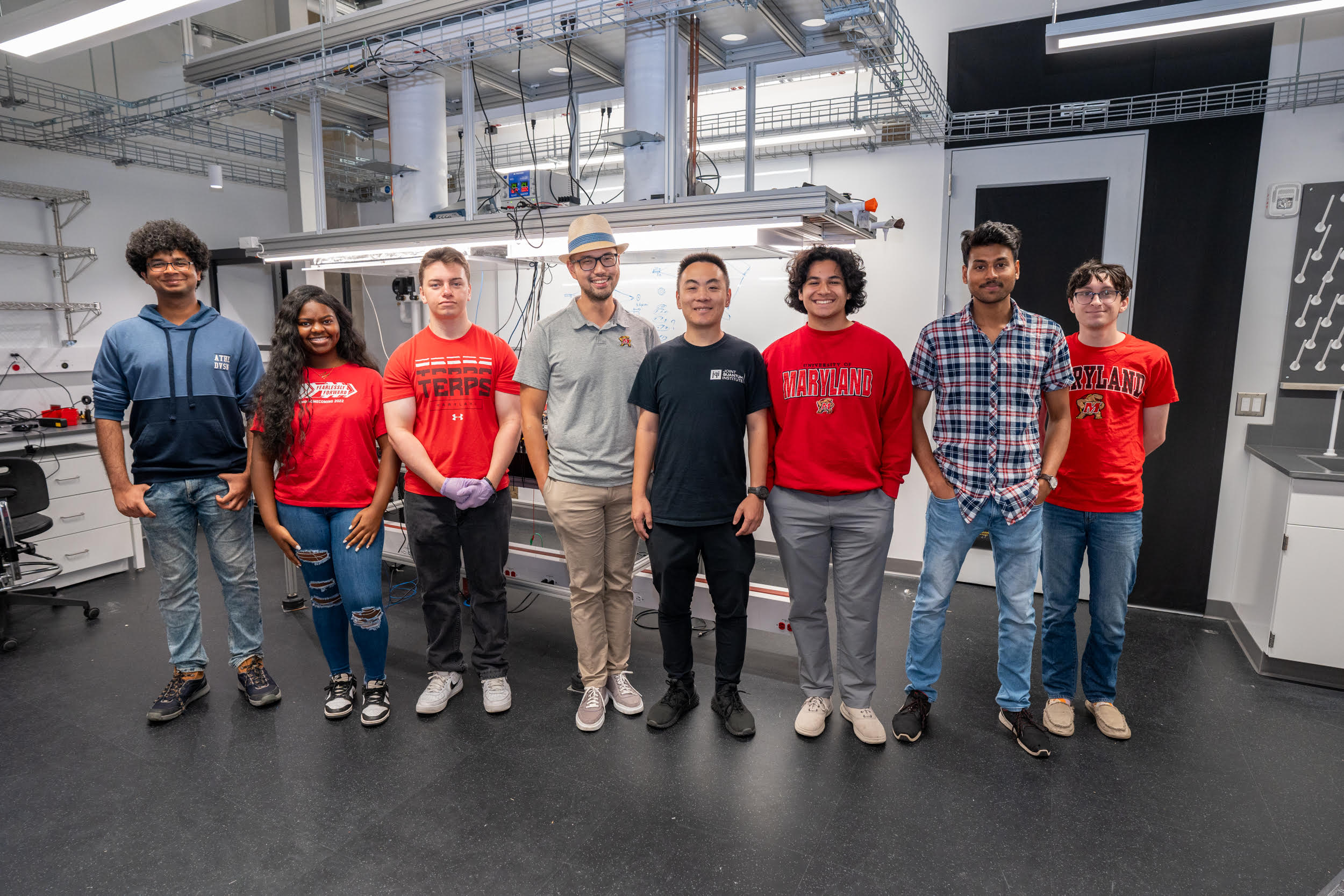
Now, Liu’s lab is pursuing two main research projects. The first is exploring chemical reactions involving only lithium. Liu selected lithium because its molecules are compatible with existing techniques for cooling to ultralow temperatures. Additionally, lithium—the third lightest element after hydrogen and helium—forms lightweight molecules. Lithium’s lightness allows theorists to calculate the behaviors that occur during chemical reactions with much higher precision than is possible for reactions involving heavier elements.
“Precise calculation, combined with accurate experimental measurements enabled by ultralow temperatures, is a recipe for better understanding the quantum dynamics of chemical reactions,” Liu says.
Liu’s second project is using an ion-neutral hybrid trap to achieve unprecedented resolution when studying a variety of chemical species—specific forms of atoms or molecules that have a characteristic energy behavior. Neutral atoms are a lot harder to cool than ions, but a hybrid trap can cool a neutral atom by ensnaring a compound that contains both it and an ion. Once the compound is cooled, the neutral atom can be gently separated and studied in cold conditions that are ideal for new discoveries.
“Essentially we’re putting a neutral inside a container that’s easy to cool and manipulate, and once we are done cooling the container, we peel off the wrapper and we reveal the cold neutral fragment that we’re actually interested in studying,” Liu says.
One of the main goals of this project is to give researchers the chance to perform experiments at ultralow temperatures with a broader range of chemical species. Liu says that chemistry is “most fun when we’re able to play with different species.”
Ultimately, Liu is working toward a deeper understanding of quantum chemistry, step by step and species by species.
“My long-term research passion is to develop more detailed understandings of how chemical reactions work,” Liu says. “When atoms in a molecule interact with each other during a chemical reaction, they play by the rules of quantum mechanics instead of Newtonian physics. My goal is to understand this level, and the way to do that is to look at chemistry—one quantum state at a time.”
This text has been adapted with permission from a story originally posted by the UMD College of Computer, Mathematical, and Natural Sciences.