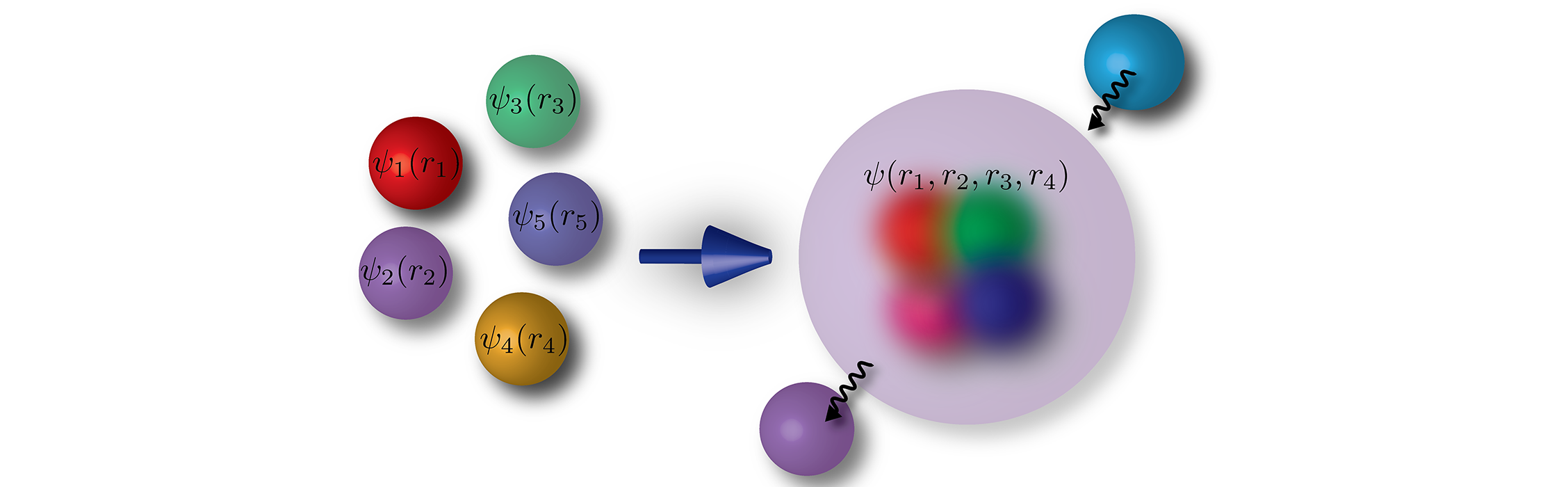
Our group aims to theoretically AND experimentally investigate various quantum properties of light-matter interaction for applications in future optoelectronic devices, quantum information processing, and sensing. Moreover, we explore associated fundamental phenomena, such as many-body physics, that could emerge in such physical systems. Our research is at the interface of quantum optics, condensed matter physics, quantum information sciences, and more recently, machine learning.
Machine learning the thermodynamic arrow of time published in Nature Physics
The mechanism by which thermodynamics sets the direction of time’s arrow has long fascinated scientists. Here, we show that a machine learning algorithm can learn to discern the direction of time’s arrow when provided with a system’s microscopic trajectory as input. The performance of our algorithm matches fundamental bounds predicted by nonequilibrium statistical mechanics.
Simons Foundation Investigator Award
The New York-based Simons Foundation will be supporting our group activities for the next five years. Simons Investigator Awards in Mathematics, Physics, Astrophysics and Computer Science support outstanding theoretical scientists in their most productive years, when they are establishing creative new research directions, providing leadership to the field and effectively mentoring junior scientists. Related JQI news.
Optical imprinting of superlattices in two-dimensional materials published in PRR
Recently, superlattice structures in two-dimensional materials, such as Moire patterns, have drawn wide interests. While most of the pre-existing methods create superlattice in passive ways, we proposed an optical method of shining circularly polarized and spatially periodic laser fields
Hafezi Wins 2020 Simons Foundation Investigator Award
JQI Fellow Mohammad Hafezi has been named a 2020 Simons Investigator in Physics by the New York-based Simons Foundation. Simons Investigator Awards in Mathematics, Physics, Astrophysics and Computer Science support outstanding theoretical scientists in their most productive years, when they are establishing creative new research directions, providing leadership to the field and effectively mentoring junior scientists.
Optical enhancement of superconductivity, highlighted in PRB
We proposed a new method for enhancing superconductivity in cuprates via melting the collective fluctuations of the competing orders.
Hafezi Named Blavatnik Award Finalist for Second Consecutive Year
For the second year in a row, JQI Fellow Mohammad Hafezi has been named a finalist of the Blavatnik National Awards for Young Scientists by the Blavatnik Family Foundation and the New York Academy of Sciences.He is among 31 of the nation’s rising stars in science who will compete for three Blavatnik National Laureate Awards in the categories of Chemistry, Physical Sciences & Engineering, and Life Sciences, and is one of 11 finalists in Physical Sciences & Engineering. Each of the three 2020 National Laureates will win $250,000—the world’s largest unrestricted prize for early-career scientists.
New Protocol Helps Classify Topological Matter
Topological materials have captured the interest of many scientists and may provide the basis for a new era in materials development. On April 10, 2020 in the journal Science Advances, physicists working with Andreas Elben, Jinlong Yu, Peter Zoller and Benoit Vermersch, including JQI Fellow Mohammad Hafezi and former JQI postdoctoral researcher Guanyu Zhu (currently a research staff member at IBM T. J. Watson Research Center), presented a new method for identifying and characterizing topological invariants on various experimental platforms, testing their protocol in a quantum simulator made of neutral atoms.
Received Google NISQ award from Google AI Quantum
From 2017, Google has been giving the award called the Google Noisy Intermediate-Scale Quantum (NISQ) award for the academic researchers who collaborated with Google's quantum computing development teams.
Synthetic Magnetism Leads Photons on a 2D Quantum Walk
Randomness governs many things, from the growth of cell colonies and the agglomeration of polymers to the shapes of tendrils that form when you pour cream into a cup of coffee.Since as early as 1905, scientists have described these seemingly unrelated phenomena in a unified way: as random walks. By imagining that individual particles or molecules are constantly taking steps in a random direction, researchers have successfully modeled many of the complexities of classical physics.More recently, scientists have brought the idea of a random walk to the quantum world, where the “walkers” can exhibit nonclassical behaviors like quantum superposition and entanglement. These quantum random walks can simulate quantum systems and may eventually be used to implement speedy quantum computing algorithms. However, this will require the walker to move in multiple dimensions (2D and higher), which has been difficult to achieve in a manner that is both practical and scalable.Quantum walks that use photons—the quantum particles of light—are particularly promising, since photons can travel long distances as energy in wave form. However, photons don’t carry an electric charge, which makes it difficult to fully control their motion. In particular, photons won’t respond to magnetic fields—an important tool for manipulating other particles like atoms or electrons. To address these shortcomings, researchers at the Joint Quantum Institute (JQI) have adopted a scalable method for orchestrating 2D quantum random walks of photons—results that were recently published in the journal Physical Review Letters. The research team, led by JQI Fellows Edo Waks and Mohammad Hafezi, developed synthetic magnetic fields in this platform that interact with photons and affect the movement of photonic quantum walkers.
Stretched Photons Recover Lost Interference
The smallest pieces of nature—individual particles like electrons, for instance—are pretty much interchangeable. An electron is an electron is an electron, regardless of whether it’s stuck in a lab on Earth, bound to an atom in some chalky moon dust or shot out of an extragalactic black hole in a superheated jet. In practice, though, differences in energy, motion or location can make it easy to tell two electrons apart.One way to test for the similarity of particles like electrons is to bring them together at the same time and place and look for interference—a quantum effect that arises when particles (which can also behave like waves) meet. This interference is important for everything from fundamental tests of quantum physics to the speedy calculations of quantum computers, but creating it requires exquisite control over particles that are indistinguishable.With an eye toward easing these requirements, researchers at the Joint Quantum Institute (JQI) and the Joint Center for Quantum Information and Computer Science (QuICS) have stretched out multiple photons—the quantum particles of light—and turned three distinct pulses into overlapping quantum waves. The work, which was published recently in the journal Physical Review Letters, restores the interference between photons and may eventually enable a demonstration of a particular kind of quantum supremacy—a clear speed advantage for computers that run on the rules of quantum physics.