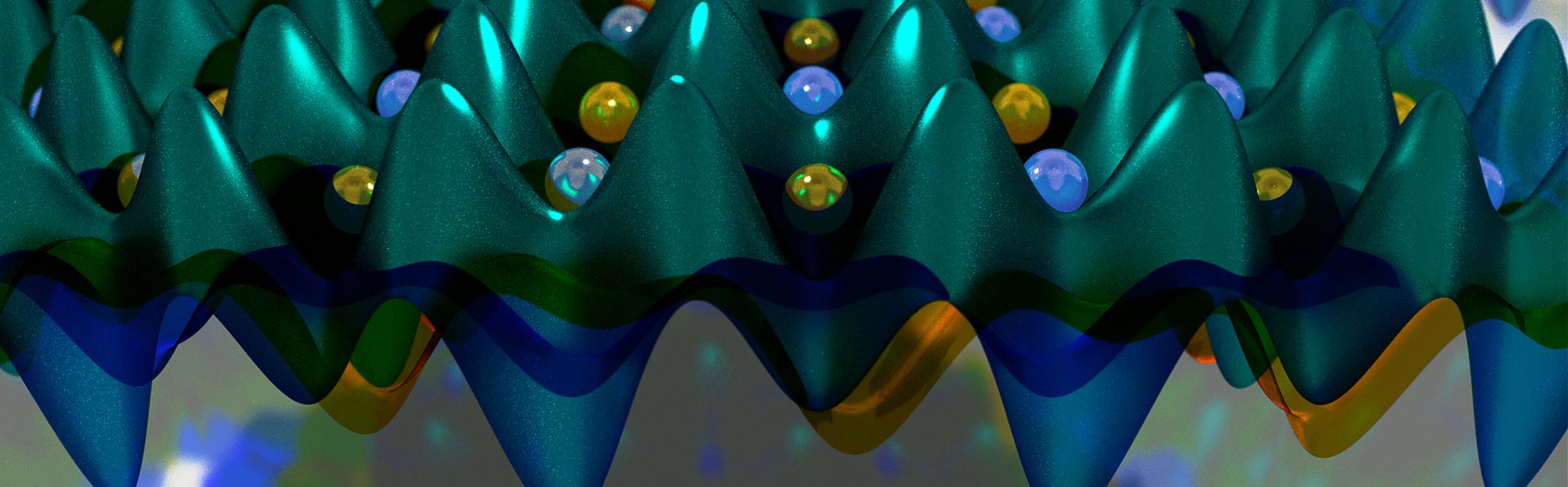
Research on ultra-cold atoms lies at the intersection of atomic physics, many-body physics, quantum optics and quantum information. Quantum physics dominates the behavior of atomic gases cooled to near absolute zero temperature, and cold trapped atoms provide an ideal experimental system for studying quantum many-body physics. Our research focuses on ultra-cold gases of Rubidium atoms and Ytterbium/Rubidium mixtures, with the goals of studying novel condensed matter systems and engineering quantum control over many-body systems, including dissipative baths.
Eric graduates!
Eric successfuly defended his thesis and is the proud owner of a new PhD!
Thomas is off to France
Thomas is headed back to France to complete his postdoc at the Inst. d'Optique working in the Browaeys group!
Two-toned light pattern creates steep quantum walls for atoms
Exotic physics can happen when quantum particles come together and talk to each other. Understanding such processes is challenging for scientists, because the particle interactions can be hard to glimpse and even harder to control. Moreover, modern computer simulations struggle to make sense of all the intricate dynamics going on in a large group of particles. Luckily, atoms cooled to near zero temperatures can provide insight into this problem.Lasers can make cold atoms mimic the physics seen in other systems—an approach that is familiar terrain for atomic physicists. They regularly use intersecting laser beams to capture atoms in a landscape of rolling hills and valleys called an optical lattice. Atoms, when cooled, don’t have enough energy to walk up the hills, and they get stuck in the valleys. In this environment, the atoms behave similarly to the electrons in the crystal structure of many solids, so this approach provides a straightforward way to learn about interactions inside real materials. But the conventional way to make optical lattices has some limitations. The wavelength of the laser light determines the location of the hills and valleys, and so the distance between neighboring valleys—and with that the spacing between atoms—can only be shrunk to half of the light’s wavelength. Bringing atoms closer than this limit could activate much stronger interactions between them and reveal effects that otherwise remain in the dark. Now, a team of scientists from the Joint Quantum Institute (JQI), in collaboration with researchers from the Institute for Quantum Optics and Quantum Information in Innsbruck, Austria, has circumvented the wavelength limit by leveraging the atoms’ inherent quantum features, which should allow atomic lattice neighbors to get closer than ever before. The new technique manages to squeeze the gentle lattice hills into steep walls separated by only one-fiftieth of the laser’s wavelength—25 times narrower than possible with conventional methods. The work, which is based on two prior theoretical proposals, was recently published in Physical Review Letters.
Sub-wavelength structured optical potentials for cold atoms
We recently demonstrated conservative optical lattices with subwavelength spatial structure. (PRL 120 083601 (2018)) The potential is based on the nonlinear optical response of three-level atoms in laser-dressed dark states. Such non-linear response is not constrained by the diffraction limit of the light generating the potential. The lattice consists of a one-dimensional array of ultranarrow barriers with widths less than 10 nm, well below the wavelength of the lattice light, physically realizing a Kronig-Penney potential.
Ancient timekeeping with a modern twist
Trey Porto, a NIST physicist and Fellow of the Joint Quantum Institute, spends his days using atoms and lasers to study quantum physics. But even outside of the lab, he views the world as one great physics problem to tackle. So one morning when he spotted some sunlight dancing across his wall, he couldn’t help but dive in and calculate its movements. He then took his project a step further and began constructing a sundial. Emily sat down with Porto to hear about his clock-making hobby and how today’s time-keeping differs from its ancient counterparts.
This episode of Relatively Certain was produced by Emily Edwards and Chris Cesare. It features music by Dave Depper and Poddington Bear. Relatively Certain is a production of the Joint Quantum Institute, a research partnership between the University of Maryland and the National Institute of Standards and Technology, and you can find it on iTunes, Google Play or Soundcloud.
"Spontaneous avalanche dephasing in large Rydberg ensembles" published
Our follow-up paper to the anamalous broadening work explores the dynamics of the black-body-induced runaway broadening process. Such broadening has serious implications for many proposals to coherently use Rydberg interactions, particularly Rydberg dressing proposals. The dephasing arises as a runaway process where the production of the first contaminant atoms facilitates the creation of more contaminant atoms. Using a pump-probe technique, we create an excess “pump” Rydberg population and probe its effect with a different “probe” Rydberg transition.
Mary is moving on to Stony Brook!
After helping build an experiment from scracth starting with an empty room, we say farewell to Mary! We wish her the best in her new adventures. Here is an early picture of the lab for old times sake.
AJ joins the group
AJ Hachtel joins the interacting photons team, working on Raman cooling of Rb.
Tsz-Chun joins the group
Tsz-Chun Tsui, a first year graduate student, joins the group and promptly passes the qualifier!
Non-linear looped band structure paper published
We published an experimental study of the stability of excited, interacting states of bosons in a double-well optical lattice in
regimes where the nonlinear interactions are expected to induce “swallowtail” looped band structure.